Heating and cooling of buildings account for roughly a third of the European Union’s total energy consumption, and nearly three quarters of the heating still comes from fossil fuels. In other words, to fulfil the EU’s target of zero impact on emissions from the building stock by 2050, it is clear that we need to switch to more sustainable technology. But how can we compare different technologies to make the most informed and sustainable choices? Here the concept of TEWI is very helpful, as a comparison of different products’ environmental impact.
The concept of TEWI
To evaluate the sustainability of a heat pump, comparing different models that rely on different technologies and then making the right choice, we need to look at and understand the concept of TEWI.
TEWI stands for Total Equivalent Warming Impact: an index that measures the global behaviour of a thermo-refrigerating unit, during its life cycle, in terms of the contribution to greenhouse emissions. Indeed, the environmental impact of a heat pump depends on a number of different factors:
- The technology used, which translates into
greater or lesser efficiency - The type and quantity of refrigerant gas used
- The operating conditions and the load profile of the heat pump
- The use of energy from renewable sources to power the heat pump
- CO2 emissions related to the production, maintenance and disposal of the unit
The TEWI index is represented by the following relation, consisting of the sum of two main components:
DGW = Direct Global Warming, which measures direct CO2 emission due to the release of greenhouse gases (refrigerant leaks).
IGW = Indirect Global Warming, which measures CO2 emissions to produce electricity or to produce thermal energy by a generator using other fuels.
As we will see below:
- the GWP of the refrigerant contributes to the DGW value;
- in the service life of a heat pump, the IGW index is an order of magnitude higher than the DGW
- the efficiency of a heat pump is more important than its GWP value
DGW – Direct Emissions
DGW=(GWP*L*M*n)+(GWP*M(1-a)) (1)
Where:
GWP = Global warming potential (specific to refrigerant gas)
L = Annual loss rate
M = Refrigerant gas charge [kg]
n = Useful life [years]
a = Recovery factor (amount of refrigerant extracted and recycled
before disposal of the unit)
- The first term, (GWP*L*M*n), evaluates the effect of emissions attributable to refrigerant leaks during the unit’s operating cycle;
- The second term, (GWP*M(1-a)), takes into account the emissions attributable to the refrigerant left in the unit and not recovered after its disposal.
From relation (1) it can be seen that the effect of direct emissions depends primarily on the GWP of the refrigerant used: the lower the GWP, the lower the (direct) CO2 emissions.
Example 1 - Direct emissions
A/W heat pump with standard energy efficiency |
High efficiency A/W heat pump |
IGW - Indirect emissions
IGW=(n*E*B)+(C*Ms)+(n*G*U) (2)
Where:
n = Useful life [years]
E = Total electricity consumption per year [kWh]
B = Intensity of CO2 emissions (CO2 released for the
production of 1 kWh of electricity) [kgCO2]
C = Conversion factor for CO2 emissions attributable to
production, maintenance and disposal. [kgCO2/kg]
MS = Mass of the full unit [kg]
G = Annual consumption of fossil energy [kWh]
U = fossil fuels conversion factor [kgCO2]
- The first term, (n*E*B), evaluates the emissions attributable to electrical consumption (operation of the unit and its auxiliary components). The total annual consumption of electricity can be obtained using energy analysis tools, taking into consideration the climatic data of the place where the unit is installed, the BIN temperature profile, the operating conditions for heating/cooling or using the load profile according to the EN14825 standard and the unit’s seasonal efficiency values (SCOP, SEER).
- On the other hand, parameter B (Intensity of CO2 emissions), relates to CO2 released for the production of electricity. The latter therefore varies considerably from country to country, depending on the mix of generation sources used: the better the mix of sources, with a higher RES (renewable energy sources) share, the lower the emission intensity index (see the diagrams below).
- The second term, (C*Ms), evaluates the emissions attributable to the production, maintenance and disposal of the unit. It is certainly a term that is not easy to estimate, however, reference models can be found in literature.
- The third term, (n*G*U) , evaluates the impact of emissions attributable to the consumption of fossil fuels. Obviously, no contribution of emissions should be taken into consideration if fossil fuels are not used and therefore only the electric heat pump is used.
Example 2 - Indirect emissions
A/W heat pump with standard energy efficiency (R410A) Nominal cooling capacity: 200 Kw 4 compressors / 2 independent circuits n =10 E = 152000 [kWh] B = 0.25 [kgCO2] C = 31.2 MS =1800 [kg] Indirect emissions = 455 tCO2e |
High efficiency A/W heat pump (“BlueBox” brand, model Zeta Sky HP R7 20.4) (R32) Nominal cooling capacity: 200 Kw 4 compressors / 2 independent circuits n =10 E = 138000 [kWh] B = 0.25 [kgCO2] C = 31.2 MS =1800 [kg] Indirect emissions = 418 tCO2e |
A practical evaluation case – three different types of air-water electric heat pumps
Boundary conditions:
- “Average” climatic conditions, for Comfort application according to EN14825;
- Useful life: 10 years;
- 3% annual loss rate, 80% recovery factor, 0.25 kgCO2 / kWh emission intensity.
Standard heat pump (R410A) |
High efficiency heat pump (R32) |
High efficiency heat pump (R290) |
![]() |
![]() |
![]() |
• HFC gas with high GWP (2088) • Standard Energy Efficiency • Hermetic scroll compressors TEWI = 507 tCO2e |
• HFC gas with average GWP (675) • High Energy Efficiency • Hermetic scroll compressors TEWI = 431 tCO2e |
• Natural Fluid: propane, GWP = 3 • High Energy Efficiency • Alternative compressors TEWI = 405 tCO2e |
The unit with the lowest impact on CO2 emissions is the heat pump that uses natural fluid (R290) as refrigerant (see “BlueBox” Titan Sky HP 20.2), with a TEWI = 405 tCO2e.
Compared to the other two units, there was a specific reduction in TEWI equal to:
- 21% compared to the standard heat pump (R410A);
- 7% compared to the R32 gas heat pump.
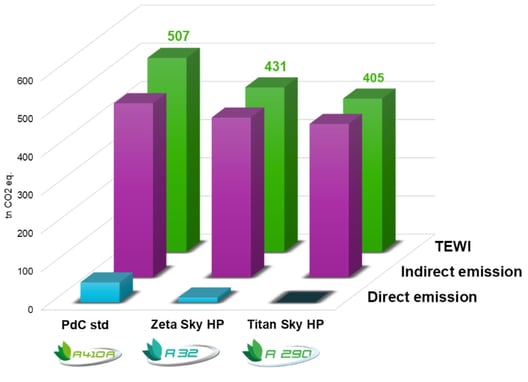
We can extend the TEWI comparison while also taking into consideration the different CO2 emission intensities for the production of electricity which, as we mentioned earlier, varies from country to country and depends on the share of renewable energy sources.
It should be noted that – given the same heat pump and other conditions – the lower the emission intensity index for electricity generation in a given country, the lower the TEWI value and the lower the greenhouse effect of a refrigeration unit.
![]() |
![]() |
Average extimation for EU 2020 |
Sweden |